Research Topics
Modern technology makes it possible today to produce structures in the size range of a few nanometers, i.e. thousands of these nanostructures fit into the diameter of a hair. For the movement of the particles in these structures, a trillionth of a second, i.e. a picosecond, is already a small eternity. On these extreme scales, completely new effects arise compared to what we know from our everyday life, because quantum physics dominates there. These effects can be studied with laser pulses and even the dynamics in the nanostructures can be controlled. Understanding them requires theoretical description and computer-aided simulations, which is the focus of our work. We consider structures made of semiconductor materials, especially so-called quantum dots, as well as metallic structures that act like antennas for visible light. The simulations allow us to predict how to control the dynamics, which is of crucial importance for applications in quantum information technology.
Reserach Areas
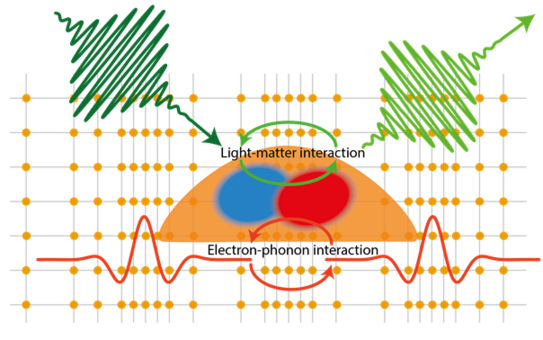
Quantum emitters produce quantum light states like single or entangled photons. Promising candidates for quantum emitters are semiconductor quantum dots. A precise optical control of the quantum dots is essential to use them in quantum technology applications. Unlike atoms, quantum dots are always coupled to phonons, which can lead to disruptive but also interesting new phenomena. Furthermore quantum dots can be confined in microcavities to study effects of semiconductor quantum optics. We study the coupled quantum dot-photon-phonon system under various aspects and using different theoretical methods. Besides quantum dots, there are several other solid-state nanostructures which we study like NV centers, defect centers in hBN or perovskite nanocrystals. All exhibit strong light-matter interaction opening up the possibilty to control.
Pushing the understand of light-matter interaction with solid-state quantum emitters, we ask ourselves: how to control the quantum dynamics? And how can we make them useful for quantum technologies? An what can we learn about quantum mechanics along they way?
Current projects
- Generation of (entangled) photons by quantum emitters (Thomas Bracht, in collaboration with AG Axt from Bayreuth, AG Rastelli from Linz and AG Weihs from Innsbruck, AG Heindel from Berlin)
- Phonon effects on optical properties of photons emitted quantum dots (Thomas Bracht, in collaboration with AG Axt from Bayreuth)
- Four-wave mixing and phonon dynamics in nanocrystal (Jan Kaspari, in collaboration with AG Akimov from TU Dortmund)
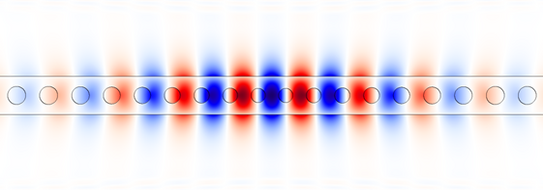
Nanostructured materials make it possible to control and specifically manipulate the properties of light on the nanoscale. An example of this is photonic crystals, in which a periodic structure means that light of a certain wavelength cannot move through the material. By intentionally creating defects, light can be spatially confined in so-called cavities. An important question here is how to excite these localized modes using a quantum emitter.
Another example of controlling light is metallic nanostructures that exhibit plasmonic resonances. This allows fields to be strongly localized, e.g., between two nanostructures, producing extremely enhanced intensity. This leads to a particularly strong light-matter interaction between the field and an emitter placed at the site of the strongest enhancement.
To study these exciting structures, we use numerical methods and also make use of existing program packages. Our focus is on understanding the light-matter interaction at the nanoscale.
Current projects
- Coupling of single emitters to cavities in photonic crystals (Jan Olthaus, Maximilian Sohr, in collaboration with AG Schuck from WWU Münster and AG Oh from Cardiff)
- Classification of photonic topological structures Jan Olthaus, in collaboration with AG Oh from Cardiff)
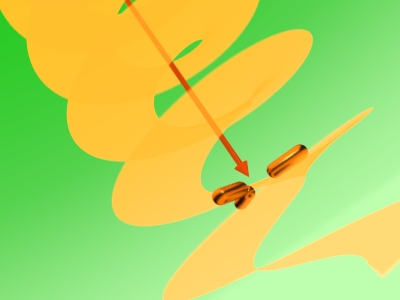
A light beam is often described by a plane wave in which the wave fronts are spatially homogeneous perpendicular to the direction of propagation. Complex light beams, on the other hand, are characterized by spatially structured wave fronts, resulting in a complex phase relationship. An example of a complex light beam is the so-called twisted light, which has a spiral wavefront as well as a phase singularity and thus a vanishing intensity on the beam axis. A twisted light beam has an additional torque, which could be exploited, for example, in communication technologies to encode information. If such a beam hits matter, different processes can be excited than with a plane wave. In this work, we are investigating on the one hand the mathematical formulation of light-matter interaction with complex light fields, and on the other hand we are performing numerical simulations to study the interaction of twisted light with metallic nanostructures exhibiting plasmonic resonances.
Current projects
- Interaction of complex light fields with plasmonic nanoantennas
- Theoretical formulation of the twisted light-matter interaction
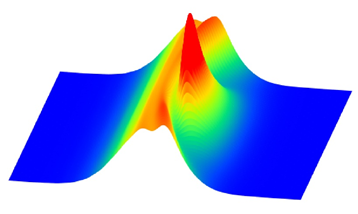
Nowadays, it is possible to produce semiconductor layers consisting of one or a few atomic layers. Examples are graphene, which has a vanishing band gap, and the so-called transition metal dichalcogenides (TMDCs) with a finite band gap. These materials are quasi-two-dimensional. In TMDCs, the potential for the conduction electrons can be changed by deformation so that a potential well is created. If electrons now move through the material, they can scatter into this potential well, releasing their excess energy in the form of phonons.
We are investigating how electrons move in these novel semiconductor materials. Due to the small scales, a quantum mechanical description is essential. We can describe the electrons as localized wave packets and show that the scattering is local. In doing so, we analyze the spatially and temporally resolved motion of the charge carriers under the influence of various interaction mechanisms.
Current projects:
- Spatially and temporally resolved charge carrier dynamics in TMDCs
- Optical measurement of spatiotemporal dynamics in semiconductors
- Signatures of phonon interaction in optical signals of TMDCs