Research Highlights
Nuclei-induced frequency focusing in quantum dots as a tool to mitigate decoherence
Electron spins localized in quantum dots are considered as qubit candidates for quantum information processing. Their interaction with the environment consisting of 104—106 nuclear spins, however, limits the coherence. Different techniques such as dynamic decoupling or dynamic nuclear polarization (DNP) can be employed to mitigate this issue. One option closely related to DNP is the so-called nuclei-induced frequency focusing effect first discovered by Greilich et al. [Science 317, 1896 (2007)]. By applying long sequences of laser pulses, the bath of nuclear spins is "trained" such that commensurate dynamics of the electron spin is favored. The result is a buildup of a coherent revival signal before the arrival of the next pulse, i.e., a partial restoration of the coherence. We have studied this effect in a series of papers by applying different approaches (semiclassical, quantum mechanical) and discovered that the pulse characteristics as well as the coupling of the nuclear spins to the applied external magnetic field crucially determine the effect's behavior and efficiency. The sensitivity to nuclear magnetic resonances (NMR), particularly studied in EPL (Europhys. Lett.) 133, 57003 (2021), raises the possibility of a novel kind of NMR spectroscopy in which the resonance frequency is imposed by the repetition rate of the laser pulses.
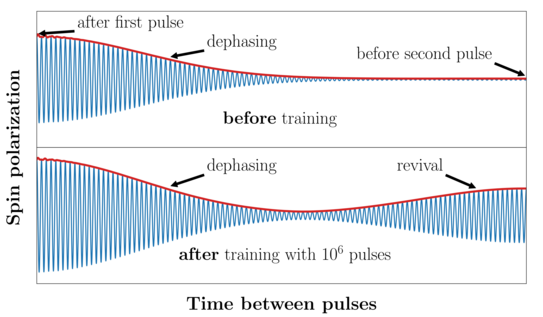
Quantum coherence from commensurate driving with laser pulses and decay
On the one hand, dissipation is detrimental to quantum coherence. It makes the quantum system relax to its thermal equilibrium which does no longer contain information, let alone quantum information based on coherence. On the other hand, a driven system without any dissipation is described by a time-dependent Hamilton operator which generates a unitary time evolution which neither destroys nor generates coherence. But we could show in SciPost Phys. 8, 40 (2020) that a combination of periodic driving and dissipation can indeed reduce the disorder of a mixed state. The entropy can be significantly reduced because states are preferred of which the dynamics is commensurate with the periodicity of the driving. This phenomenon has been observed already in experiments on spins in quantum dots and spin waves. Commensurability as a tool for tailoring quantum systems and states appears as a promising route for coherent control.
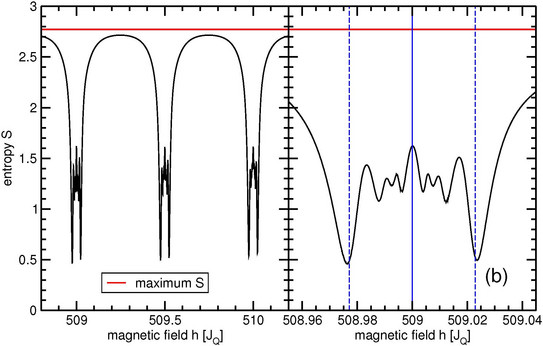
Resonant spin amplification in Faraday geometry
There are various techniques that allow one to characterize the spin dynamics in semiconductor nanostructures. Popular examples are the Hanle effect, time-resolved pump-probe spectroscopy, spin-noise spectroscopy, and measurements of the polarization recovery in combination with the spin-inertia technique. One of the most basic parameters characterizing the spin dynamics is the g factor, which is often anistropic in semiconductor nanostructures. Its transverse component can be measured very precisely when a magnetic field is applied in Voigt geometry by means of the resonant spin amplification effect, where the spin polarization is resonantly amplified whenever the Larmor precession period is a multiple of the laser repetition period. In Phys. Rev. Research 1, 033189 (2019), we have suggested that this effect can also appear under certain conditions if small longitudinal magnetic fields (Faraday geometry) are applied. It enables the direct measurement of the longitudinal g factor, which was previously accessible only by indirect methods. Soon after the original proposal, the first experimental realization of this novel effect for an ensemble of quantum dots was presented in Phys. Rev. B 103, L201301 (2021). In a close collaboration with our experimental colleagues from E2, we supported their measurements by a detailed and accurate modeling of the experiment. Thanks to the great agreement achieved between experiment and theory, we were able to guide the experiment towards better visbility conditions.
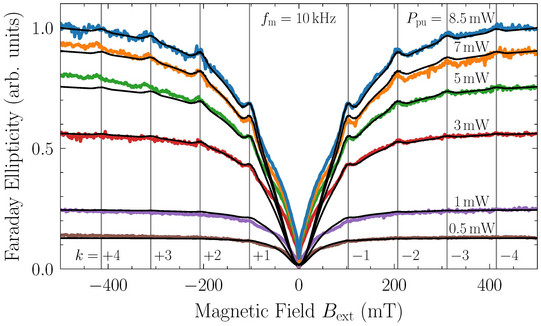
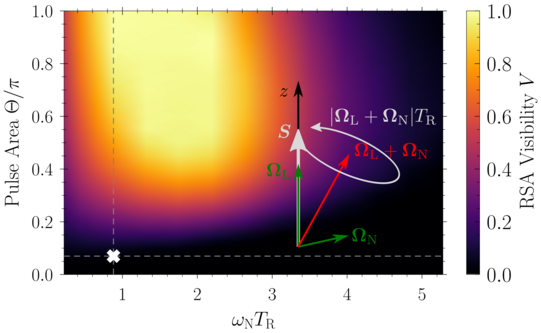
Topological magnonics
Topological properties play an increasingly important role in future research and technology. This also applies to the field of topological magnetic excitations, which has recently become a very active and broad field. The idea of is to use spin waves (magnons) to transmit, store, and process information while exploiting the fact that topological properties display a certain robustness against perturbations such as imperfetions and disorder. Just recently, we have contributed to the 2021 Magnonics Roadmap published in J. Phys. Condens. Matter 33, 413001 (2021) and we have also written a perspective article about topological magnetic excitations in EPL (Europhys. Lett.) 132, 20003 (2020). Sitting at the cutting-edge of research in the growing field of magnonics, we have suggested the usage of topological magnon bands for magnonics in Phys. Rev. B 99, 174412 (2019), where we study edge modes in the ferromagnetic Shastry-Sutherland lattice that can be put to use in magnonic devices. Since one of the main challenges in this field is to identify more magnetic systems with non-trivial topology, we have also identified a class of potential candidates in this work.
Research: Correlated solid state systems with prominent quantum effects
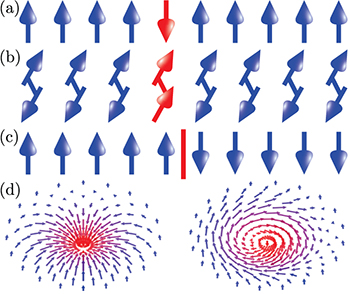
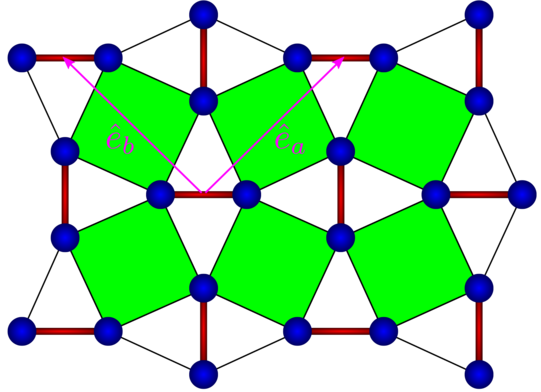
Classification and characterization of nonequilibrium Higgs modes in unconventional superconductors
Recent findings of new Higgs modes in unconventional superconductors require a classification and characterization of the modes allowed by nontrivial symmetry of the superconducting gap. In Nat. Commun. 11, 287 (2020), we have developed a theory for a tailored nonequilibrium quantum quench to excite all possible oscillation symmetries of a superconducting condensate. It turns out that both a finite momentum transfer and quench symmetry allow for an identification of the resulting Higgs oscillations that serve as a fingerprint for the ground state gap symmetry. By resorting to group theory, a classification scheme of the Higgs oscillations and the quench symmetry is provided. It allows us to distinguish between different symmetries of the superconducting condensate.
Research: Correlated solid state systems with prominent quantum effects
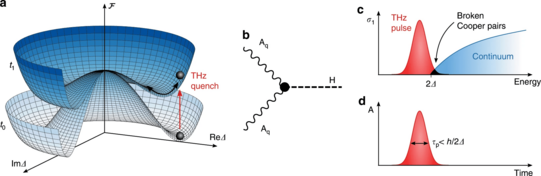